Aromatic compounds absorb UV light because of their cyclic structure and the delocalization of π electrons. When UV light hits these compounds, it excites their π electrons, causing shifts from bonding π orbitals to anti-bonding π* orbitals. This absorption typically happens in the 200-400 nm range due to their unique energy gaps, which are influenced by the degree of conjugation in the molecule. The specific wavelengths absorbed depend on the compound's structure. Understanding these properties not only sheds light on their behavior but also opens up fascinating insights into their applications and biological significance.
Key Takeaways
- Aromatic compounds have delocalized π electrons due to their cyclic structure, allowing them to absorb UV light effectively.
- The electronic shifts of π electrons occur from bonding π orbitals to higher energy anti-bonding π* orbitals under UV exposure.
- The presence of conjugated systems lowers the energy gap between bonding and anti-bonding orbitals, facilitating UV absorption.
- Each aromatic compound has a unique maximum absorption wavelength (λmax) that reflects its specific electronic structure and degree of conjugation.
- Functional groups on aromatic compounds can modify their UV absorption characteristics and enhance their biological functions, such as photoprotection.
Structure of Aromatic Compounds

Aromatic compounds, like benzene, possess a unique cyclic structure that features alternating single and double bonds, which creates a conjugated π system.
In these aromatic rings, each carbon atom contributes one p-orbital, allowing for the delocalization of the π electrons across the entire ring. This delocalization enhances the stability of the molecule through resonance, making it less reactive than non-aromatic compounds.
The specific arrangement of electrons results in distinct energy gaps between bonding and anti-bonding orbitals. Because of this, aromatic compounds can absorb UV light at characteristic wavelengths, which is essential for their identification and analysis in various chemical applications.
Understanding the structure helps you appreciate the unique properties of these compounds.
Mechanism of UV Absorption
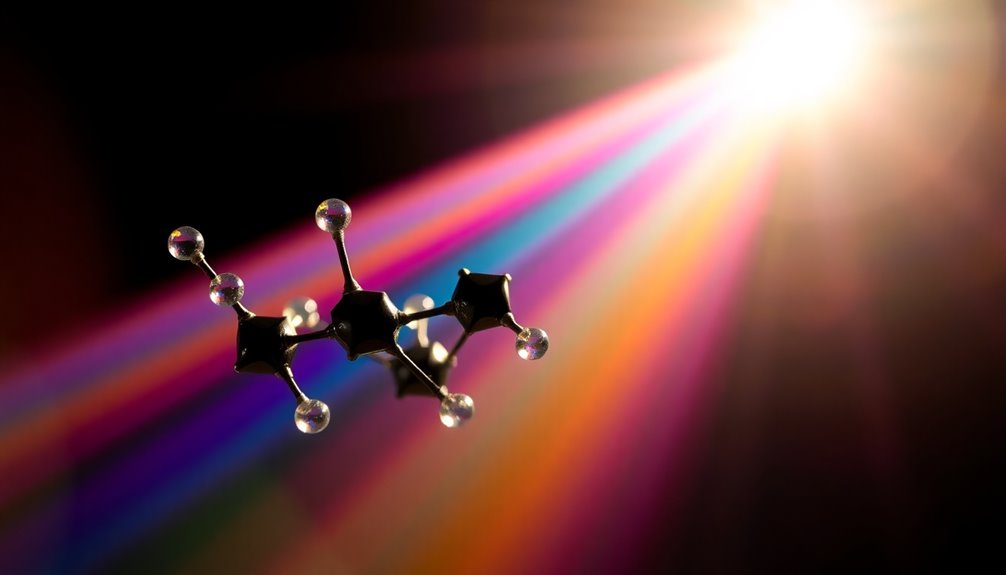
When UV light interacts with aromatic compounds, it triggers electronic shifts of π electrons. These changes occur as the π electrons move from bonding π orbitals to higher energy anti-bonding π* orbitals.
The energy associated with these changes corresponds to specific wavelengths absorbed, typically ranging from 200 to 400 nm. Aromatic compounds, due to their conjugated π bonds, exhibit a lower energy gap between these orbitals, allowing them to absorb UV light at longer wavelengths compared to non-aromatic compounds.
Each aromatic compound has a unique maximum absorption wavelength (λmax), which reflects its distinct electronic structure. This characteristic behavior in UV absorption is influenced by the extent of delocalization, enhancing stability and resulting in unique spectral patterns.
Importance of Conjugation
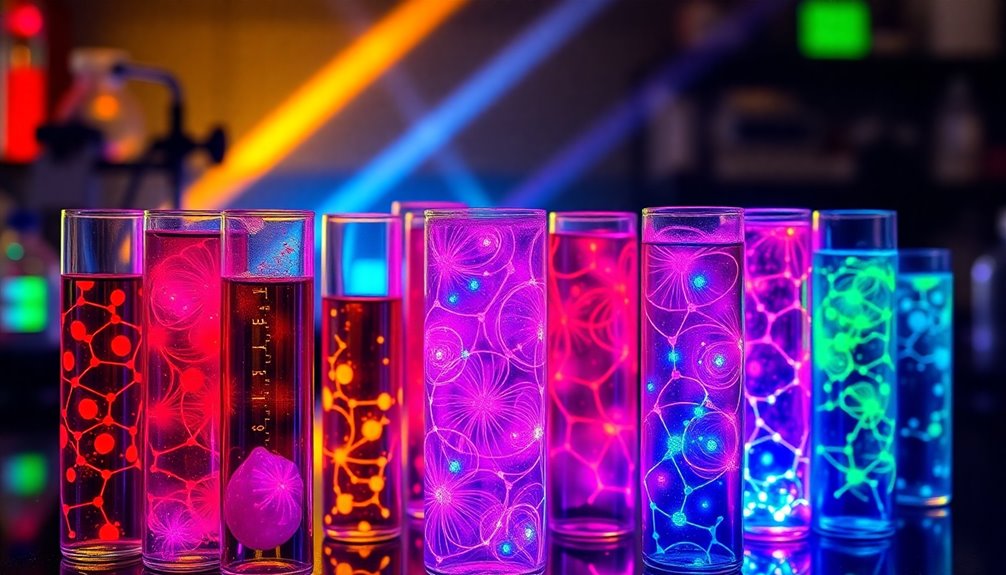
The interaction of UV light with aromatic compounds highlights the significance of conjugation in determining their absorption properties.
When you look at conjugated systems, you'll see that the overlap of p-orbitals across adjacent double bonds creates delocalized electrons. This overlap effectively lowers the energy gap between bonding and anti-bonding orbitals, allowing for electronic shifts at longer wavelengths.
As the extent of conjugation increases, these compounds become more stable and are more likely to absorb UV light. This absorption typically occurs within the 200-400 nm range, which is detectable by UV spectroscopy.
Consequently, understanding conjugation is essential for predicting how aromatic compounds will interact with UV light, impacting various applications in chemistry and materials science.
Role of Electron Delocalization
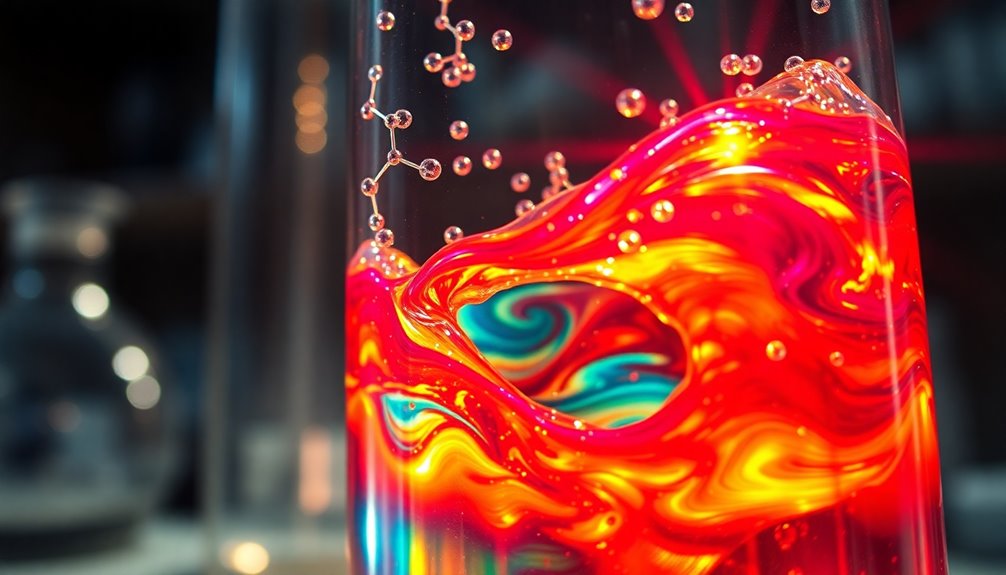
When you explore aromatic compounds, you'll notice that electron delocalization enhances their stability.
This sharing of electrons across multiple atoms lowers the energy needed for electronic shifts. As a result, these compounds can absorb UV light more efficiently, allowing for unique spectral properties.
Enhanced Stability Through Delocalization
Although aromatic compounds might seem simple, their enhanced stability primarily stems from the delocalization of π electrons across their ring structures. This delocalization lowers the overall energy of the system, creating a stable electronic configuration that makes these compounds less reactive than non-aromatic ones.
The presence of conjugated π bonds further contributes to this stability by allowing greater electron delocalization. In aromatic compounds, the energy gap between bonding π and anti-bonding π* orbitals is reduced, enabling easier excitation of electrons by UV light.
As a result, increased delocalization leads to distinctive absorption peaks in UV spectra, which are vital for identifying and analyzing these fascinating molecules. Understanding this stability offers insights into their unique properties.
Lower Energy Transition Requirements
Because aromatic compounds feature extensive electron delocalization within their conjugated π systems, they require lower energy for electronic changes compared to non-aromatic molecules. This electron delocalization reduces the energy gap between bonding and anti-bonding orbitals, enabling shifts from π bonding to π anti-bonding orbitals at longer wavelengths.
For instance, benzene, with three conjugated π bonds, absorbs UV light at approximately 254 nm due to these shifts. The more extensive the conjugation in an aromatic compound, the lower the energy required for absorption, allowing it to capture UV light effectively.
Additionally, substituents or functional groups can modify these energy levels, further enhancing or shifting the UV absorption characteristics of these compounds. Such properties underscore the unique behavior of aromatic compounds in UV absorption.
Absorption Spectrum Characteristics
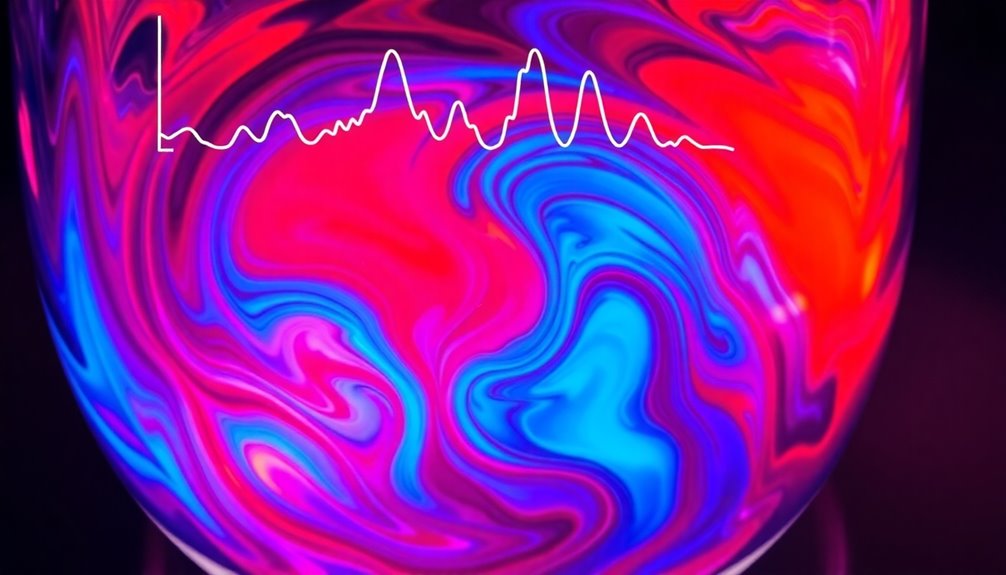
When you look at the absorption spectrum of aromatic compounds, you'll notice that electronic changes of π electrons play a key role in their interaction with UV light.
The presence of conjugated π systems not only lowers the energy gap but also shifts the absorption peaks to longer wavelengths.
Electronic Transitions in Aromatics
Aromatic compounds play a fascinating role in the absorption of UV light, primarily due to the electronic shifts of their π electrons. These electronic changes occur when π electrons move from bonding π orbitals to higher energy anti-bonding π* orbitals. The energy gap between these orbitals directly corresponds to specific UV wavelengths, giving each aromatic compound its unique absorption characteristics.
For instance, benzene showcases maximum absorption around 254 nm, thanks to its stable conjugated π system. As you increase conjugation in aromatic systems, the energy gap decreases, allowing for the absorption of longer wavelengths, like those seen in anthracene.
Substituents on aromatic rings can further modify the absorption spectrum, impacting the observed λmax values by altering the energy levels of the π and π* orbitals.
Role of Conjugation
The relationship between conjugation and UV absorption in aromatic compounds is fundamental to understanding their spectral characteristics.
Conjugated π systems play a significant role in lowering the energy gap between bonding and anti-bonding orbitals. This reduction allows for electronic shifts at longer wavelengths.
As you explore different aromatic compounds, you'll notice that the extent of conjugation directly correlates with the wavelength of UV light absorbed. For instance, benzene's fully conjugated system with six π electrons absorbs maximally around 254 nm, while more complex systems like anthracene absorb at even longer wavelengths.
The π to π* shifts in these conjugated systems are responsible for their distinct UV absorption characteristics, highlighting the importance of conjugation in influencing the compounds' electronic properties and reactivity.
Absorption Peak Analysis
Absorption peaks in the UV spectrum serve as a window into the structural characteristics of aromatic compounds. When you analyze these peaks, you'll notice that they typically fall between 200-300 nm, reflecting the energy gap between bonding π orbitals and anti-bonding π* orbitals.
The degree of conjugation in an aromatic system influences the energy for electron shifts; as conjugation increases, you'll observe longer wavelengths absorbed. Additionally, different substituents can shift absorption peaks, providing insights into electron distribution and molecular structure.
Tryptophan and UV Absorption
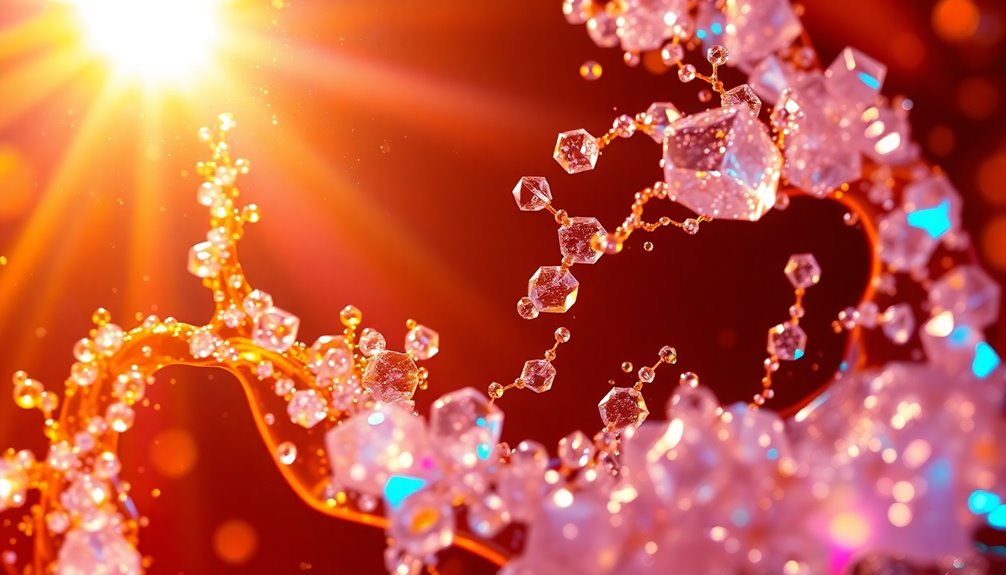
When studying UV light interactions in proteins, you can't overlook tryptophan, an aromatic amino acid that absorbs UV light at around 280 nm.
Its indole side chain features a conjugated π system, allowing electronic shifts from bonding π orbitals to higher energy anti-bonding orbitals. This unique structure, with double bonds and lone pair electrons, enhances tryptophan's UV absorption capabilities.
- Tryptophan's absorption is critical for protein dynamics.
- It helps track protein folding and interactions.
- Its UV absorption contributes to photoprotection.
In the energy diagram, you can see how the presence of sigma bonds complements this process, making tryptophan essential for understanding biochemical research and the stability of proteins in biological systems.
Biological Significance of Aromatics
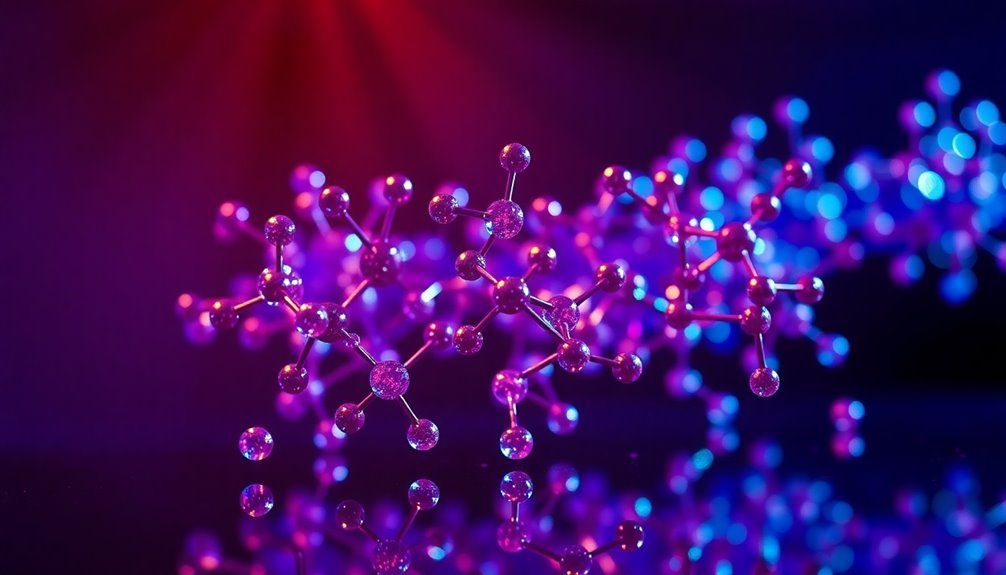
Aromatic compounds play an essential role in various biological processes beyond just their UV absorption properties.
You'll find that compounds like tryptophan and phenylalanine absorb UV light due to their conjugated π systems, which allow for significant electronic alterations. These aromatic amino acids are important in protein analysis and help monitor enzyme activities and protein folding dynamics.
Their unique functional groups also contribute to the photoprotection of proteins, absorbing harmful UV radiation and preserving cellular integrity.
Additionally, aromatic structures are imperative for synthesizing neurotransmitters such as serotonin, derived from tryptophan.
Applications in Spectroscopy
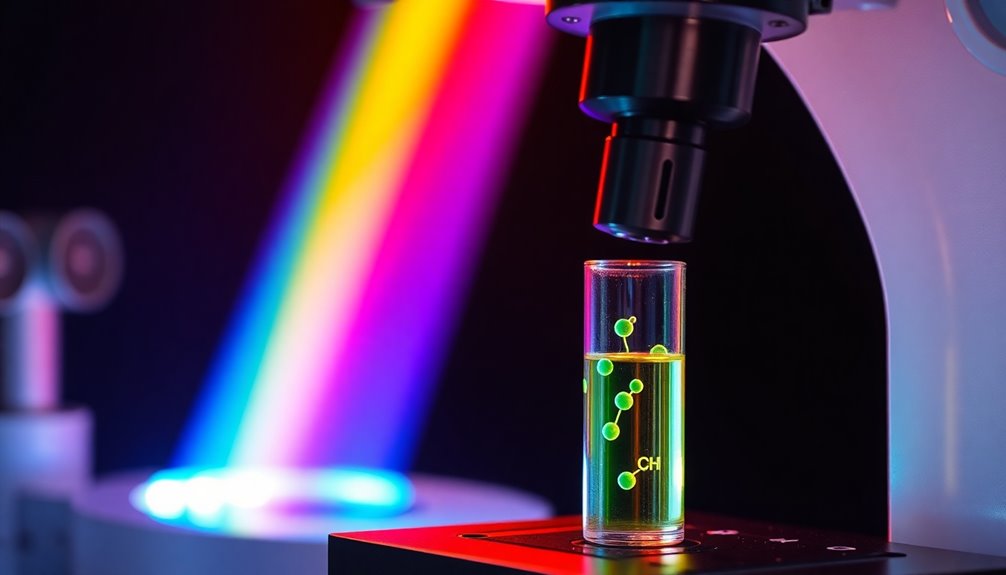
While exploring the applications of spectroscopy, you'll find that aromatic compounds are invaluable due to their distinctive UV absorption characteristics. Their conjugated bonds allow for electron shifts that produce unique absorption spectra, making them essential for qualitative analysis.
Here are some key applications:
- UV spectroscopy: Identifies aromatic compounds by their characteristic λmax, typically around 250-300 nm.
- Chromatographic methods: Enhances detection limits, aiding in the separation of complex mixtures.
- Biochemical research: Facilitates the study of protein interactions and biological dynamics through UV absorption data.
Color Perception and Complementary Colors
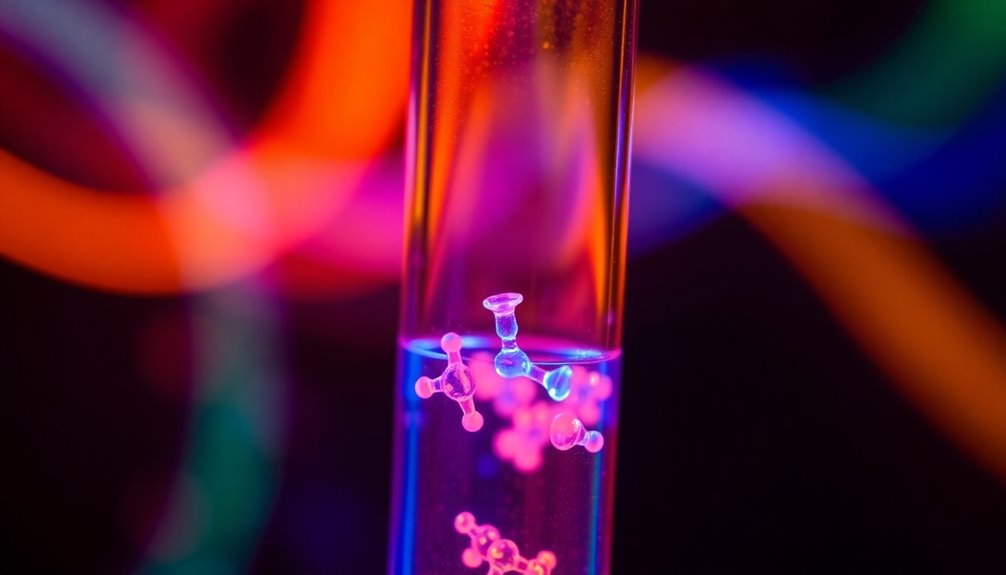
When you observe the color of an aromatic compound, you're actually seeing the complementary color of the wavelengths it doesn't absorb.
For example, beta-carotene absorbs blue light, making it appear orange to your eyes.
This relationship between absorption and color perception reveals how different wavelengths can create a diverse palette of colors.
Complementary Color Relationships
Understanding complementary color relationships is essential for grasping how we perceive color in various compounds. When a compound absorbs light at specific wavelengths, the color we see is its complementary color. For instance, if a compound absorbs blue light, it appears yellow.
- The color wheel shows complementary colors opposite each other, like red and cyan.
- Beta-carotene absorbs light in the blue to cyan region, resulting in an orange appearance.
- Phenolphthalein changes color based on pH, turning magenta in alkaline conditions due to its absorption of visible light.
Wavelength Absorption Effects
Aromatic compounds reveal fascinating interactions with UV light that directly affect color perception. When these compounds absorb specific wavelengths, it's due to the excitation of π electrons jumping to higher energy anti-bonding orbitals.
The energy gaps between the π and π* orbitals dictate which wavelengths are absorbed. For instance, compounds absorbing UV light in the blue region may appear orange to you because of complementary color relationships.
The unique electronic shifts in these aromatic compounds determine not just the wavelengths absorbed but also the colors reflected back to your eye. Understanding these relationships is essential in fields like dye chemistry and material science, where the color characteristics play a significant role in product applications.
Challenges in Molecular Analysis
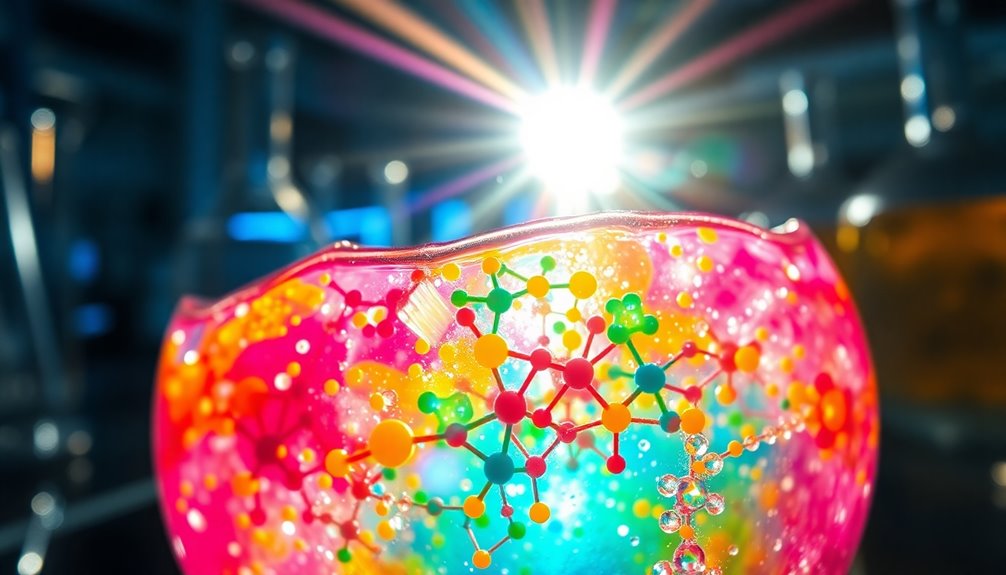
Analyzing aromatic compounds presents several challenges that can complicate molecular analysis. Their resonance structures create multiple canonical forms, which can obscure the actual electron distribution and distort spectral data.
Additionally, the presence of overlapping electronic alterations in UV spectra results in congested absorption peaks, making it tough to differentiate compounds in a mixture.
- Variations in substituents can alter UV absorption characteristics.
- Solvent polarity influences absorption properties, affecting consistency.
- Canonical forms may lead to unexpected shifts in absorption wavelengths.
To achieve accurate analysis, you'll need careful calibration and interpretation of UV spectra, considering these factors to navigate the complexities inherent in aromatic compounds.
Frequently Asked Questions
Why Does Benzene Absorb UV Light?
Benzene absorbs UV light because its π electrons can be excited to higher energy anti-bonding orbitals when exposed to radiation.
You'll notice that the maximum absorption wavelength is around 254 nm, which aligns with the energy gap between bonding and anti-bonding π orbitals.
The stability and resonance of benzene's structure play an essential role, allowing it to effectively absorb UV light, making it useful in applications like chromatography for identifying compounds.
Why Do Aromatic Compounds Fluoresce?
Aromatic compounds fluoresce because they can absorb energy and undergo electronic changes.
When you shine UV light on them, the π electrons get excited to higher energy states. As these electrons return to their ground state, they release energy in the form of visible light, creating fluorescence.
The efficiency of this process depends on factors like the compound's structure and environmental conditions, which can influence how much light is emitted and at what wavelength.
Why Do Aromatic Amino Acids Absorb Light?
Aromatic amino acids absorb light like a sponge soaking up water. Their unique structure, featuring conjugated π systems, enables them to shift electrons from bonding to anti-bonding orbitals when exposed to UV light.
Tryptophan, for instance, has a peak absorption around 280 nm, thanks to its indole side chain. This ability to absorb light is essential for studying proteins, revealing insights into their dynamics and interactions in biological systems.
Why Do Some Molecules Absorb UV Light?
Some molecules absorb UV light because their electrons can be excited to higher energy levels when they encounter specific wavelengths. This absorption occurs when there's a significant energy difference between the ground and excited states.
Factors like molecular structure, bond type, and electron configuration play essential roles. If a molecule has conjugated systems or specific functional groups, it's more likely to absorb UV light, enhancing its reactivity and interaction with light.
Conclusion
To sum up, aromatic compounds are fascinating for their ability to absorb UV light due to their unique structures and electron delocalization. Did you know that over 60% of the known organic compounds are aromatic? This statistic highlights their prevalence and importance in both nature and science. Understanding why these compounds absorb UV light not only aids in molecular analysis but also enhances our appreciation for the vibrant colors and complex behaviors of the world around us.