Aromatic compounds are fascinating structures you'll find in many aspects of chemistry. They consist of cyclic carbon rings, like benzene, featuring alternating single and double bonds. This unique arrangement allows for resonance, which boosts their stability. You'll encounter various types, including monosubstituted compounds, polycyclic aromatic hydrocarbons, and even those with heteroatoms. They play vital roles in industries like pharmaceuticals and fragrance, while also appearing in natural processes. Their special properties make them essential in reactions and biological systems. If you're curious about their applications and significance, there's much more you'll uncover!
Key Takeaways
- Aromatic compounds are cyclic structures with planar carbon rings and alternating single and double bonds, exemplified by benzene.
- They follow Hückel's rule, with π electrons equal to 4n + 2, ensuring aromaticity and stability through resonance.
- Aromatic compounds can be monosubstituted, polycyclic, or heterocyclic, and can be derived from fossil fuels, nature, or industrial processes.
- They typically undergo electrophilic substitution reactions, with reactivity influenced by substituents that determine the regioselectivity of the reactions.
- Aromatic compounds have various applications, including in the textile, fragrance, and pharmaceutical industries, as well as in environmental remediation.
Definition of Aromatic Compounds
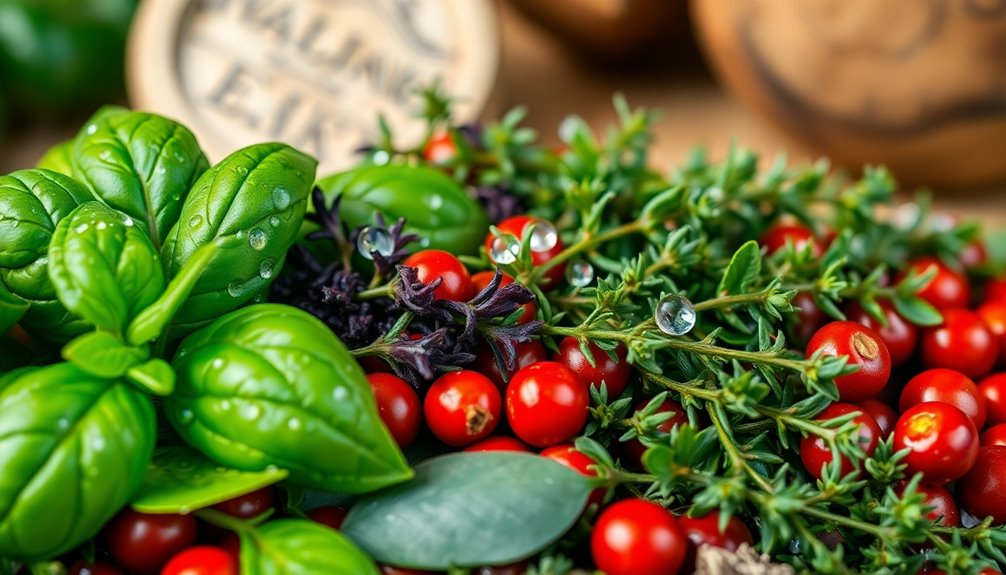
Aromatic compounds are fascinating structures in organic chemistry. Defined as cyclic structures, they contain at least one planar carbon ring with alternating single and double bonds. A prime example is benzene, the simplest aromatic compound, characterized by its hexagonal shape and equal bond lengths due to resonance.
For a compound to be aromatic, it must adhere to Hückel's rule, which states that the number of π electrons must equal 4n + 2. This unique arrangement leads to enhanced stability and lower reactivity compared to non-aromatic compounds.
Additionally, the presence of substituents on the aromatic ring can influence its properties and reactivity, producing various structural isomers like ortho, meta, and para derivatives.
Structure and Stability

When you look at the structure of aromatic compounds like benzene, you'll notice a planar ring with alternating single and double bonds.
This arrangement leads to resonance, allowing π electrons to be delocalized across the ring, which greatly boosts stability.
As a result, aromatic compounds are less reactive than their aliphatic counterparts, thanks to their unique resonance energy.
Benzene Ring Structure
The benzene ring is a fascinating structure that showcases the unique properties of aromatic compounds. It consists of six carbon atoms arranged in a planar hexagonal configuration, featuring alternating single and double bonds, known as resonance. This arrangement leads to equal bond lengths of approximately 1.39 Å.
Each carbon atom is sp² hybridized, allowing for the delocalization of π electrons above and below the ring, creating a continuous loop of electron density. Benzene adheres to Hückel's rule, possessing six π electrons, which contributes to its aromatic stability and lower reactivity.
Instead of undergoing addition reactions, the benzene ring primarily engages in substitution reactions, preserving its aromaticity while facilitating the introduction of various substituents, enhancing its versatility in organic chemistry.
Resonance and Stability
Six key factors contribute to the resonance and stability of aromatic compounds. First, the delocalization of π electrons across a planar configuration leads to equal bond lengths among carbon-carbon bonds. Hückel's rule, which states that aromatic compounds must contain (4n + 2) π electrons, confirms their stability; for instance, benzene with 6 π electrons is a classic example. The resonance stabilization results in lower heats of combustion and hydrogenation compared to non-aromatic compounds, indicating greater stability. In addition, the presence of delocalized electrons reduces reactivity, making aromatic compounds less prone to disruptive addition reactions.
Factor | Description |
---|---|
Resonance | Delocalization of π electrons |
Hückel's Rule | (4n + 2) π electrons requirement |
Planar Configuration | Essential for effective p orbital overlap |
Bond Lengths | Equal lengths due to resonance |
Reactivity | Lower due to stable electron distribution |
Types of Aromatic Compounds

Aromatic compounds come in various types, each with unique characteristics that influence their behavior and applications. You might encounter monosubstituted compounds like toluene, where a hydrogen atom is replaced by a methyl group on the benzene ring.
Polycyclic aromatic hydrocarbons (PAHs) feature multiple fused aromatic rings, with naphthalene being a prime example. Heterocyclic aromatic compounds include at least one heteroatom, such as in pyridine and furan. Non-benzenoid aromatic compounds, like cyclopropenyl cation, showcase aromaticity without being benzene derivatives.
Additionally, you can categorize aromatic compounds by structural features like monocyclic, bicyclic, and tricyclic forms. These classifications not only highlight their diversity but also hint at their potential applications and reactivity in various chemical contexts.
Chemical Properties
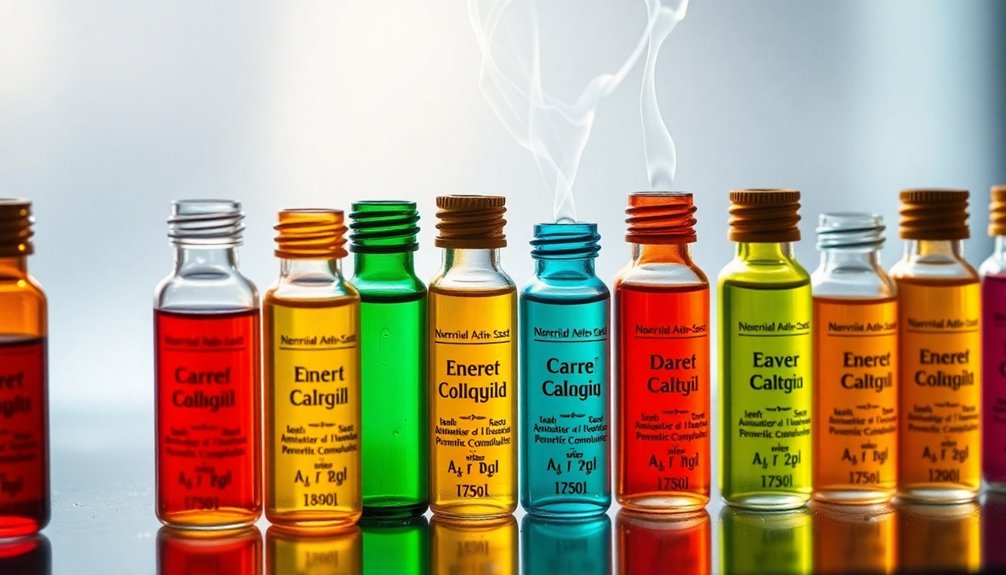
Understanding the types of aromatic compounds sets the stage for exploring their chemical properties. Aromatic compounds exhibit unique electronic properties due to resonance stabilization, making them less reactive than non-aromatic compounds.
Instead of addition reactions, they typically undergo electrophilic substitution reactions, which preserve the aromatic ring during chemical transformations. The π electrons in these systems are tightly held, contributing to their stability.
Substituents on the aromatic ring play a significant role in determining reactivity; activators tend to favor ortho-para substitution, while deactivators lead to meta substitution. This interplay influences the pathways and products formed during synthesis, showcasing the nuanced behavior of aromatic compounds in various reactions.
Understanding these properties is essential for effective manipulation in organic chemistry.
Reactions Involving Aromatic Compounds

When you explore reactions involving aromatic compounds, you'll find that hydrogenation transforms arenes into saturated compounds, altering their reactivity.
Additionally, electrophilic substitution mechanisms allow electrophiles to replace hydrogen atoms while keeping the aromatic structure intact.
Understanding these processes is key to manipulating the chemistry of aromatic compounds effectively.
Hydrogenation of Arenes
Hydrogenating arenes transforms these stable compounds into saturated cycloalkanes, fundamentally altering their chemical properties. This process involves adding hydrogen to the aromatic ring, which requires a catalyst like palladium, platinum, or Raney nickel.
Through hydrogenation, you disrupt the stable π electron system, leading to the loss of aromaticity and the formation of saturated compounds. You can control the degree of hydrogenation to obtain specific products, such as converting 1-naphthol into a mixture of decalin-ol isomers.
This reaction is vital in synthetic chemistry, enhancing the reactivity of aromatic compounds or creating intermediates for further transformations. Remember, reaction conditions like temperature and pressure greatly influence the efficiency of the hydrogenation process, with higher pressures favoring complete saturation.
Electrophilic Substitution Mechanisms
Electrophilic aromatic substitution (EAS) reactions allow you to replace hydrogen atoms on an aromatic ring with various electrophiles while preserving the compound's aromaticity. The mechanism typically involves the formation of a sigma complex, a high-energy intermediate that disrupts the aromatic π system.
Common EAS reactions include nitration, sulfonation, and halogenation, each utilizing specific electrophiles like nitronium ions (NO₂⁺) and halogen cations (Br⁺ or Cl⁺).
The directing effects of substituents on the aromatic ring are vital, categorizing them as activators (ortho-para directors) or deactivators (meta directors). This regioselectivity influences the formation of multiple isomers, depending on the positions of existing substituents.
Understanding these mechanisms is essential for manipulating aromatic compounds in organic synthesis.
Applications in Industry

Aromatic compounds play an important role in various industrial applications, serving as essential feedstocks for the production of fundamental chemicals and materials.
Benzene, toluene, and xylene, collectively known as BTX, are crucial in the chemical industry, forming the basis for plastics and synthetic fibers.
In pharmaceuticals, these compounds act as key intermediates, with many drugs featuring aromatic structures that enhance their effectiveness.
When it comes to dyes, aromatic compounds like aniline and azo dyes deliver vibrant colors used in textiles and inks.
In the fragrance industry, they contribute to perfumes and food additives, enriching sensory experiences.
Additionally, they serve as solvents in industrial processes and play a role in bioremediation, helping bacteria degrade aromatic pollutants in contaminated environments.
Sources of Aromatic Compounds

While many aromatic compounds are derived from fossil fuels, you'll find that their sources are diverse, ranging from natural processes to industrial production.
Here are some key sources of aromatic compounds you should know:
- Fossil Fuels: Coal and petroleum are primary sources, with aromatic hydrocarbons like benzene, toluene, and xylene extracted during refining.
- Natural Occurrences: Volcanic activity and forest fires release aromatic compounds into the environment.
- Natural Products: Essential oils and various plant-derived substances are rich in aromatic compounds, contributing unique fragrances.
- Industrial Processes: Millions of tons of these compounds are produced annually for applications in chemicals, plastics, and pharmaceuticals.
Understanding these sources helps you appreciate the widespread presence and significance of aromatic compounds in our world.
Importance in Biological Systems

As you explore the significance of aromatic compounds in biological systems, you'll discover that they play essential roles in various processes.
Important amino acids like phenylalanine and tryptophan are crucial for protein synthesis. Many hormones, including estrogen and testosterone, feature aromatic rings that enhance their biological activity and receptor interactions. Additionally, these hormones can influence neurotransmitter release, contributing to mood regulation. Furthermore, certain essential oils derived from aromatic plants can also impact mood and emotional well-being.
Benzene serves as a precursor to significant molecules, such as dopamine, which regulates mood and behavior. While polycyclic aromatic hydrocarbons (PAHs) can be harmful, some bacteria can metabolize them, showcasing their dual role in the environment.
In addition, aromatic compounds contribute to plant secondary metabolites like flavonoids and alkaloids, aiding in plant defense and offering health benefits through their antioxidant properties. Furthermore, certain herbal extracts have been studied for their potential to provide anti-aging effects, highlighting the intersection of aromatic compounds and natural health benefits.
Frequently Asked Questions
What Defines an Aromatic Compound?
To define an aromatic compound, you need to look for a cyclic structure with at least one planar carbon ring. This ring should have alternating single and double bonds, creating a stable arrangement through resonance.
You'll find that all carbon atoms are sp² hybridized, contributing to its planarity. Additionally, the compound must meet Hückel's rule, ensuring there are 4n + 2 π electrons for aromatic stability.
Substituents can further affect its reactivity.
How Do You Identify Aromatic Compounds?
Identifying aromatic compounds is like uncovering hidden treasures in a chemical world!
You'll look for a cyclic structure with alternating single and double bonds. Check for resonance stabilization and make sure it follows Hückel's rule—4n + 2 π electrons.
If you spot delocalized π electrons, you're on the right track. Remember, these compounds typically have distinctive odors and are nonpolar, setting them apart from their polar counterparts.
Happy exploring!
What Is the Definition of Aromatic and Non-Aromatic Compounds?
Aromatic compounds are cyclic structures with alternating single and double bonds, containing (4n + 2) π electrons, making them stable due to resonance.
In contrast, non-aromatic compounds don't fit these criteria, either lacking the required π electrons or having non-planar structures that hinder effective overlap of p orbitals.
Recognizing these differences helps you understand the distinct chemical behaviors and reactivities of these compounds in various reactions and applications.
What Is Aromatic and Aliphatic?
Aromatic compounds have a unique cyclic structure with alternating double and single bonds, giving them resonance and stability. You'll find that they follow Huckel's rule, featuring (4n + 2) π electrons.
In contrast, aliphatic compounds consist of linear or branched structures without aromatic rings and can be saturated or unsaturated. The key difference lies in their stability and reactivity; aromatic compounds are generally less reactive than their aliphatic counterparts, favoring substitution reactions.
Conclusion
To conclude, aromatic compounds are like the backbone of many chemical processes, providing stability and reactivity that shape various industries and biological systems. Their unique structure allows them to engage in fascinating reactions, making them invaluable in everything from pharmaceuticals to fragrances. Understanding these compounds not only deepens your knowledge of chemistry but also highlights their significance in our everyday lives. By appreciating their role, you can better grasp the intricate connections within the world of science.