Aromatic compounds are intriguing structures you'll encounter in chemistry. They have a cyclic arrangement of carbon atoms, forming at least one planar ring. These compounds feature alternating single and double bonds, which grants them stability through a phenomenon called resonance. The simplest example is benzene, which contains six carbon atoms in a hexagonal shape. You might also come across various types, such as naphthalene or heterocyclic compounds. Their unique properties make them essential in many industries, from pharmaceuticals to dyes. There's much more to explore about their behavior and applications that you might find fascinating.
Key Takeaways
- Aromatic compounds are cyclic structures with alternating single and double bonds, exhibiting resonance stabilization.
- They must adhere to Huckel's rule, possessing (4n + 2) π electrons for aromaticity.
- Benzene is the simplest aromatic compound, characterized by a planar hexagonal structure.
- Aromatic compounds are widely used in pharmaceuticals, textiles, and as solvents in various industries.
- Their stability and reactivity differ significantly from non-aromatic compounds, influencing their chemical behavior.
Definition of Aromatic Compounds

Aromatic compounds are fascinating structures in organic chemistry, defined by their unique cyclic arrangement. To be classified as aromatic, a compound must contain at least one planar carbon ring with alternating single and double bonds, resulting in a distinct stability known as aromaticity.
Benzene, the simplest aromatic compound, showcases this with its hexagonal structure and resonance stabilization from delocalized π electrons. To meet the criteria for aromaticity, the number of π electrons in the ring must follow Huckel's rule, expressed as 4n + 2.
Aromatic compounds can be monocyclic like benzene or polycyclic like naphthalene, and they exhibit unique properties, such as reduced reactivity and the ability to engage in electrophilic substitution reactions.
Structure of Benzene

Benzene's structure is a striking hexagonal plane, where each carbon atom connects to the others with alternating bonds.
You'll find that the delocalized pi electrons create resonance stability, making benzene uniquely resistant to reactions that typically affect similar compounds.
This arrangement not only defines its aromatic character but also influences its chemical behavior and stability.
Planar Hexagonal Structure
When you examine the structure of benzene, you'll find a planar hexagonal arrangement of six carbon atoms, each bonded to a hydrogen atom, creating the chemical formula C6H6.
This benzene ring features sp² hybridized carbon atoms, which results in a bond angle of 120 degrees, ensuring that all carbon atoms remain in the same plane.
The alternating sigma bonds and resonance contribute to the stability of aromatic compounds, giving rise to six equivalent carbon-carbon bonds, each with a bond order of 1.5.
The equal bond lengths of approximately 1.39 Å distinguish benzene from aliphatic compounds.
This unique structure not only enhances stability but also exemplifies the characteristics of planar rings in aromatic chemistry.
Delocalized Pi Electrons
The stability of the planar hexagonal structure arises from the delocalized π electrons that circulate freely across the six carbon atoms in benzene. Each carbon is sp² hybridized, promoting effective overlap of π orbitals. This delocalization results in equal bond lengths of approximately 1.39 Å between carbon-carbon bonds.
Characteristic | Value | Significance |
---|---|---|
Carbon Atoms | 6 | Forms benzene rings |
Delocalized π Electrons | 6 | Confirms aromatic compound status |
Bond Length | 1.39 Å | Shorter than single bonds |
Reactivity | Lower | Resists addition, favors substitution |
These delocalized π electrons enhance benzene's stability, making it less reactive than alkenes, which is key in defining aromatic compounds.
Resonance Stability Factors
Although benzene may appear to have alternating single and double bonds, its true structure is defined by resonance, which greatly influences its stability.
The benzene ring consists of six carbon atoms, each bonded to one hydrogen atom. This arrangement allows for the delocalization of six π electrons, resulting in resonance stabilization.
Instead of distinct C—C bonds, all bond lengths are equal at approximately 1.39 Å, reflecting a bond order of 1.5. This unique electron delocalization contributes to benzene's low reactivity and remarkable stability as an aromatic compound.
According to Huckel's rule, the presence of these 6 π electrons (where n=1) confirms benzene's aromaticity, further highlighting its exceptional stability compared to other compounds.
Types of Aromatic Compounds

Aromatic compounds are fascinating due to their diverse classifications, which include monoaromatic, polycyclic aromatic hydrocarbons (PAHs), and heterocyclic aromatic compounds.
Each type exhibits unique characteristics:
- Monocyclic aromatic compounds: These have a single benzene ring, like benzene or toluene.
- Polycyclic aromatic compounds (PAHs): These consist of multiple fused benzene rings, such as naphthalene and anthracene.
- Heterocyclic aromatic compounds: These include one or more heteroatoms in the ring, like pyridine and furan.
- Aromatic nature: All types benefit from resonance structures, allowing delocalization of π electrons, enhancing stability.
Understanding these classifications helps you appreciate the diverse roles aromatic compounds play in chemistry and everyday life.
Huckel's Rule and Aromaticity
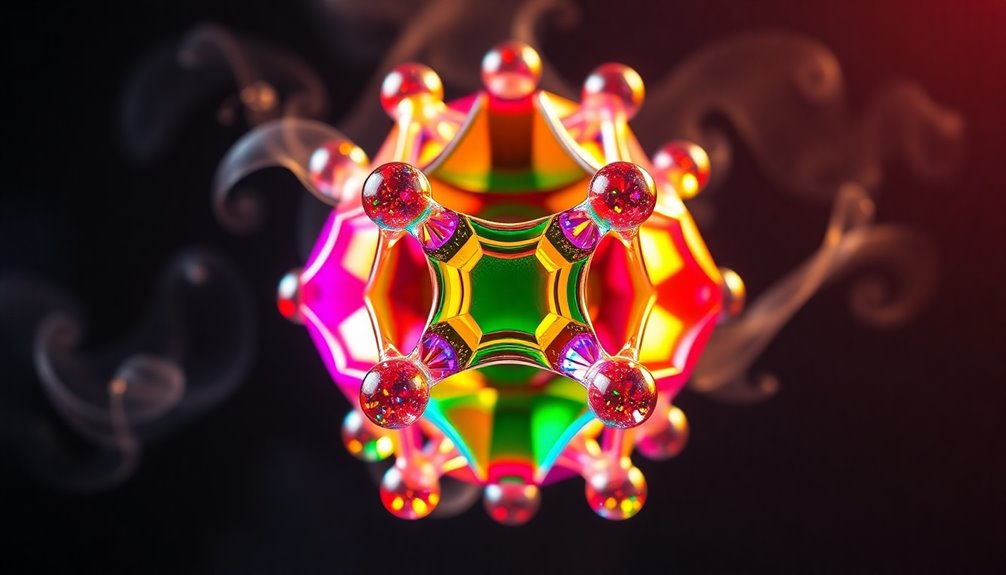
How can we determine if a compound truly possesses aromaticity? You'll want to refer to Huckel's rule, which states that aromatic compounds contain a planar, cyclic structure with (4n + 2) π electrons, where n is a non-negative integer.
For example, benzene, with 6 π electrons (n=1), is a classic aromatic compound because it adheres to this rule. Similarly, naphthalene and propylbenzene also satisfy Huckel's rule, each having 10 π electrons.
In contrast, compounds like cyclobutadiene, which has 4 π electrons, are classified as antiaromatic since they don't meet the (4n + 2) criterion.
Understanding Huckel's rule is essential for predicting the stability and reactivity of these cyclic compounds in various chemical applications.
Chemical Properties of Aromatic Compounds
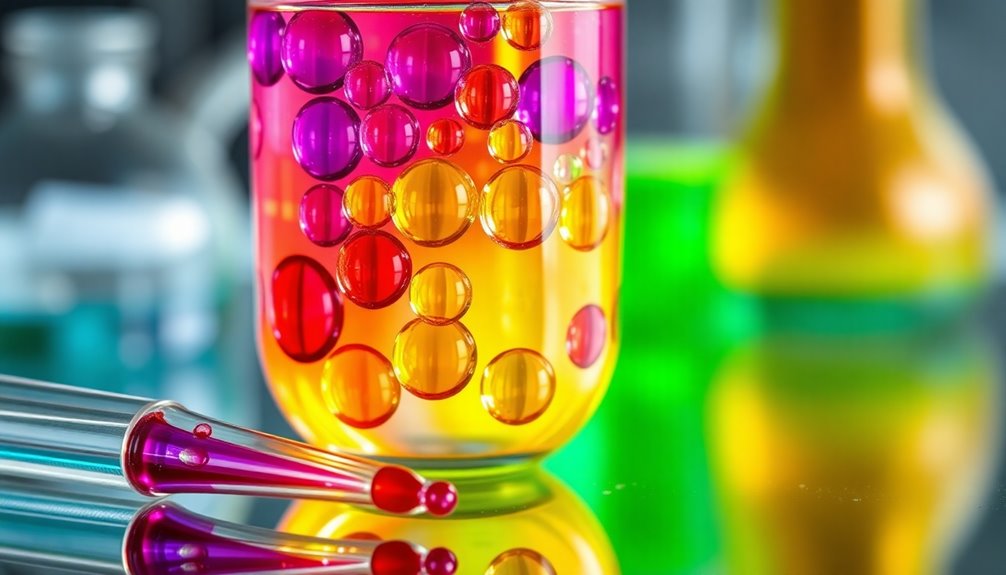
When you explore the chemical properties of aromatic compounds, you'll notice their unique stability comes from resonance, making them less reactive than non-aromatic counterparts.
They typically favor electrophilic substitution reactions instead of addition reactions, thanks to their delocalized π electrons.
This behavior not only influences their reactivity but also defines their overall chemical character.
Stability and Reactivity
Understanding the stability and reactivity of aromatic compounds is essential for grasping their chemical behavior. The unique stability arises from resonance, where π electrons are delocalized across the aromatic ring. This leads to bond lengths that are equivalent and lower heats of combustion.
Here are some key points to remember:
- Aromatic compounds follow Huckel's rule, needing (4n + 2) π electrons for stability.
- The strong holding of π electrons makes them less reactive than non-aromatic compounds.
- Substitution reactions dominate due to this stability.
- Electrophilic substitution reactions allow the aromatic ring to act as a nucleophile, maintaining its aromaticity.
Electrophilic Substitution Reactions
Aromatic compounds exhibit distinctive chemical properties due to their stability, particularly in undergoing electrophilic substitution reactions. In these reactions, a hydrogen atom on the aromatic ring is replaced by an electrophile, forming a sigma complex before restoring aromaticity. The regioselectivity is key, influenced by substituents already on the ring.
Type of Group | Effect on Substitution | Preferred Position |
---|---|---|
Electron-Donating | Activates the ring | Ortho or Para |
Electron-Withdrawing | Deactivates the ring | Meta |
Examples | -OH, -OCH₃ | |
-NO₂, -CF₃ |
With the right conditions, like catalysts and elevated temperatures, you can achieve high yields in these fascinating reactions.
Substitution Reactions
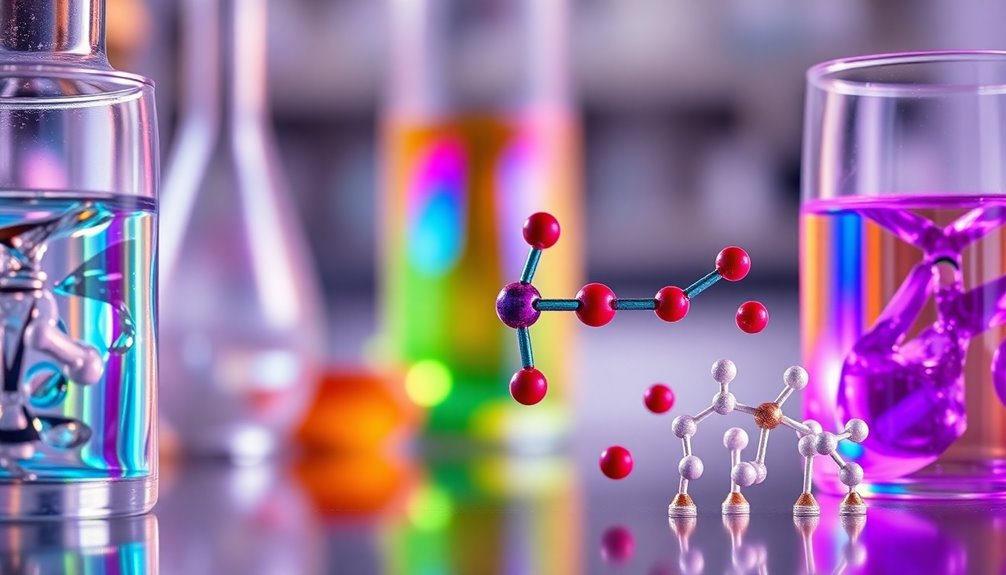
Substitution reactions play an essential role in modifying aromatic compounds to create a variety of derivatives. You'll encounter two primary types: electrophilic aromatic substitution (EAS) and nucleophilic aromatic substitution (NAS). Each type allows you to replace hydrogen atoms with different substituents.
Here are some key points to remember:
- EAS involves an electrophile reacting with the aromatic ring.
- NAS requires a nucleophile and a good leaving group, often needing electron-withdrawing groups.
- Activating groups speed up substitution, while deactivating groups can slow it down.
- The position of substituents can be classified as ortho, meta, or para, affecting reactivity.
Understanding these processes will help you manipulate aromatic compounds effectively!
Hydrogenation Processes

When you add hydrogen to unsaturated compounds, hydrogenation transforms them into saturated forms, such as converting benzene into cyclohexane.
This reaction typically requires a catalyst like palladium, platinum, or Raney nickel to speed up the process.
As you proceed with hydrogenation, the aromatic compounds lose their aromaticity, leading to significant changes in their chemical properties, including reactivity and boiling points.
This transformation is essential in various industrial applications, especially in producing cycloalkanes, which serve as solvents and intermediates in chemical synthesis.
The degree of hydrogenation can vary based on reaction conditions such as pressure, temperature, and the presence of additives or solvents, resulting in different products and applications.
Dearomatization Reactions
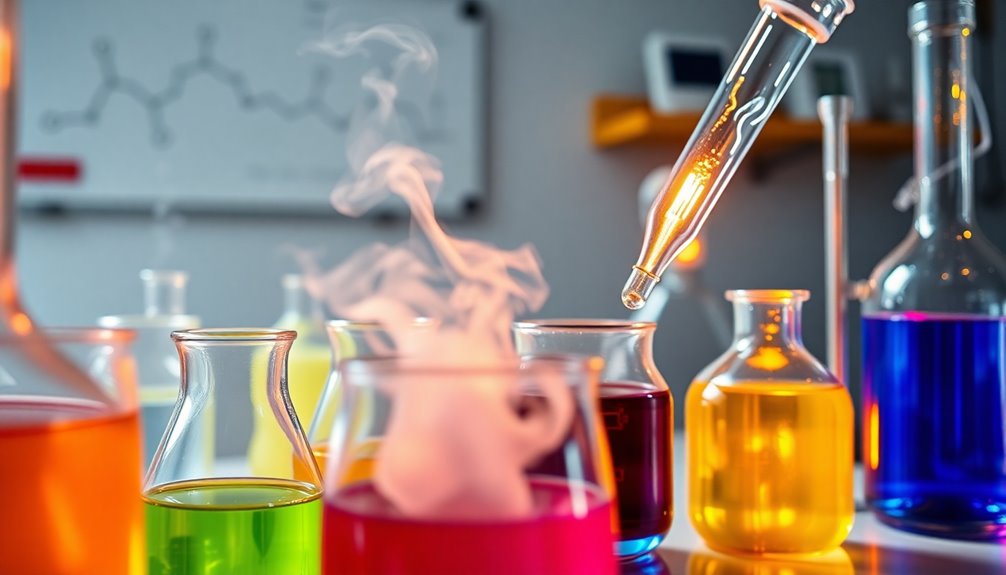
Dearomatization reactions play a crucial role in transforming aromatic compounds into more reactive and diverse structures.
These reactions involve losing aromaticity, leading to new chemical properties. You might find the following methods particularly interesting:
- Birch reduction: Reduces aromatic rings to non-aromatic cyclohexadienes.
- Hydrogenation: Adds hydrogen to aromatic compounds, creating saturated ring structures.
- Increased reactivity: The loss of aromaticity changes how these compounds behave in subsequent reactions.
- Synthetic chemistry utility: These transformations help create complex molecules from simple starting materials.
Applications in Industry

Aromatic compounds are integral to various industries, particularly in pharmaceuticals, where many essential drugs derive from benzene derivatives like aspirin and ibuprofen.
They're vital in the dye industry, acting as key intermediates for vibrant colors in textiles, plastics, and inks.
In industrial applications, aromatic hydrocarbons such as benzene, toluene, and xylene (BTX) are effective solvents widely used in paints, coatings, and adhesives.
Additionally, the production of plastics and synthetic fibers heavily relies on these compounds, with polystyrene and polyethylene terephthalate (PET) being prime examples.
Aromatic compounds also enhance fragrances and flavorings, with vanillin and eugenol finding extensive use in food and cosmetics, showcasing their versatility and significance across multiple sectors.
Environmental Impact and Sustainability

When you consider the environmental impact of aromatic compounds, their biodegradation and toxicity come to the forefront.
These substances can pose serious risks to ecosystems and human health due to their persistence and potential for bioaccumulation.
Understanding these factors is essential for developing sustainable practices in their production and use.
Biodegradation of Aromatic Compounds
Although they're essential to various industrial processes, aromatic compounds, especially polycyclic aromatic hydrocarbons (PAHs), pose serious environmental challenges due to their persistence and toxicity.
Fortunately, microbial biodegradation offers a natural solution. Specific bacteria and fungi can effectively break down these harmful compounds, reducing environmental contamination.
Here are some key points about microbial biodegradation of aromatic compounds:
- Certain bacteria, like Pseudomonas and Mycobacterium, can achieve degradation rates of up to 90%.
- Complex metabolic pathways, such as ortho and meta-cleavage, are involved in the breakdown process.
- Enzymes like dioxygenases play a pivotal role in initiating biodegradation.
- Bioremediation strategies are being developed to enhance the efficiency of these natural processes.
This approach contributes considerably to sustainability efforts in environmental management.
Toxicity and Environmental Risks
Given their widespread presence in industrial emissions, aromatic compounds, particularly polycyclic aromatic hydrocarbons (PAHs), pose serious toxicity and environmental risks.
These environmental pollutants, including benzene, toluene, and xylene, can contaminate air, soil, and water, leading to significant health issues, including cancer due to their carcinogenic properties.
The degradation of these harmful compounds is essential for bioremediation, as specific bacteria can metabolize them, reducing their toxicity and overall environmental impact.
Regulatory measures, like the EPA's guidelines, aim to limit exposure to these hazardous substances and monitor their levels.
Additionally, researchers are exploring sustainable production methods that utilize bioengineering to synthesize aromatic compounds, reducing reliance on petroleum sources and mitigating associated environmental risks.
Frequently Asked Questions
What Is Meant by Aromatic Compounds?
When you think about aromatic compounds, you're looking at a special class of chemicals that have unique cyclic structures.
They contain at least one carbon ring with alternating single and double bonds, which gives them remarkable stability.
You'll find that these compounds often have distinct odors and don't mix well with water, instead dissolving in organic solvents.
Understanding their properties can help you appreciate their applications in various fields, from chemistry to everyday products.
How Do You Identify Aromatic Compounds?
Imagine uncovering a hidden treasure in a garden of molecules!
To identify aromatic compounds, look for their unique cyclic structure with alternating single and double bonds, which creates a dance of delocalized electrons.
Check if they follow Huckel's rule—those π electrons should fit the 4n + 2 formula.
Finally, notice their charming stability, often reflected in lower heats of combustion.
With practice, you'll become an expert in spotting these delightful chemical gems!
What Is Aromatic and Aliphatic?
Aromatic compounds have a unique cyclic structure with alternating double and single bonds, making them stable due to resonance. They follow Huckel's rule, which involves having (4n + 2) π electrons.
In contrast, aliphatic compounds consist of straight or branched carbon chains and lack this resonance stabilization.
You'll find that aromatic compounds usually have distinct odors and lower reactivity, while aliphatic ones tend to be more reactive and can be saturated or unsaturated.
What Is a Simple Aromatic Compound?
A simple aromatic compound usually features a hexagonal ring structure made up of six carbon atoms, like benzene.
You'll notice that these compounds often have alternating double bonds, which provide them with unique stability due to delocalized electrons.
When you substitute hydrogen atoms with other groups, you create derivatives like toluene or phenol.
These compounds are more stable and less reactive than aliphatic ones, and they often emit distinctive odors.
Conclusion
In summary, aromatic compounds play an important role in both nature and industry, weaving their way into countless applications. Their unique structures and properties make them indispensable, like the hidden threads in a tapestry that bind everything together. As we harness these compounds, it's essential to reflect on their environmental impact and aim for sustainable practices. By balancing innovation with responsibility, we can enjoy the benefits of aromatic compounds while preserving our planet for future generations.