To determine which compounds are aromatic, you'll want to identify cyclic structures with delocalized π electrons. Benzene is a classic example, meeting Hückel's rule with six π electrons. Phenol also qualifies due to its cyclic shape and resonance. In contrast, compounds like cyclohexane and methane lack the necessary cyclic or conjugated structures, making them non-aromatic. Remember, anti-aromatic compounds like cyclobutadiene are unstable because they don't satisfy Hückel's rule. If you're curious about more examples and their applications in chemistry, you might find what's next quite enlightening.
Key Takeaways
- Aromatic compounds must be cyclic, planar, and have a fully conjugated system of p orbitals for resonance stability.
- They must satisfy Hückel's rule, containing 4n + 2 π electrons for aromaticity.
- Benzene is a classic example, exhibiting six π electrons and significant resonance energy.
- Phenol is also aromatic, containing a hydroxyl group and fulfilling the criteria for stability and reactivity.
- Non-aromatic compounds lack cyclic structure or π bonds, while anti-aromatic compounds have 4n π electrons, leading to instability.
Definition of Aromatic Compounds
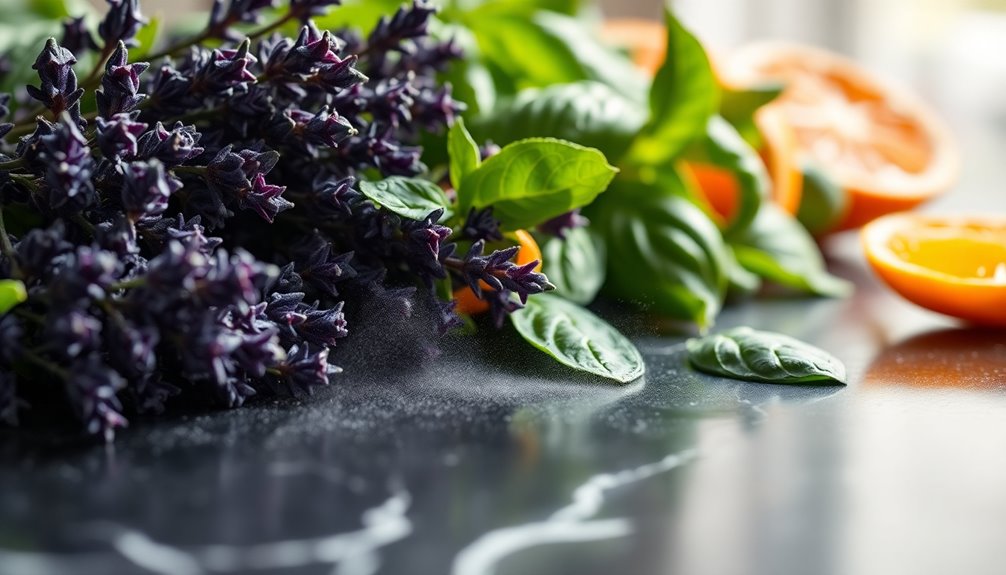
Aromatic compounds are fascinating because they possess unique properties that set them apart from other cyclic structures. Defined by a cyclic arrangement, these compounds feature a delocalized π electron system that contributes to their exceptional stability.
To be classified as aromatic, a compound must be planar and meet Hückel's rule, which specifies that it must have 4n + 2 π electrons, where n is a non-negative integer. This delocalization of π electrons occurs through a fully conjugated system of p orbitals, allowing for resonance that enhances stability.
However, not all cyclic compounds qualify; those lacking planarity or adequate conjugation fall into the categories of non-aromatic or anti-aromatic. Understanding these criteria is essential for identifying aromatic compounds.
Criteria for Aromaticity

To determine whether a compound is aromatic, several key criteria must be met.
First, the compound needs to be cyclic, allowing for a continuous loop of p orbitals.
Next, it must be planar; this planarity is essential for the effective overlap of p orbitals, enabling the delocalization of pi electrons.
Additionally, an aromatic compound must possess an uninterrupted cloud of pi electrons, which requires a fully conjugated system.
Finally, according to Huckel's rule, the compound should contain an odd number of pairs of pi electrons, specifically following the formula 4n + 2, where n is a non-negative integer.
These criteria collectively contribute to the stability and unique properties of aromatic compounds.
Example Analysis of Benzene
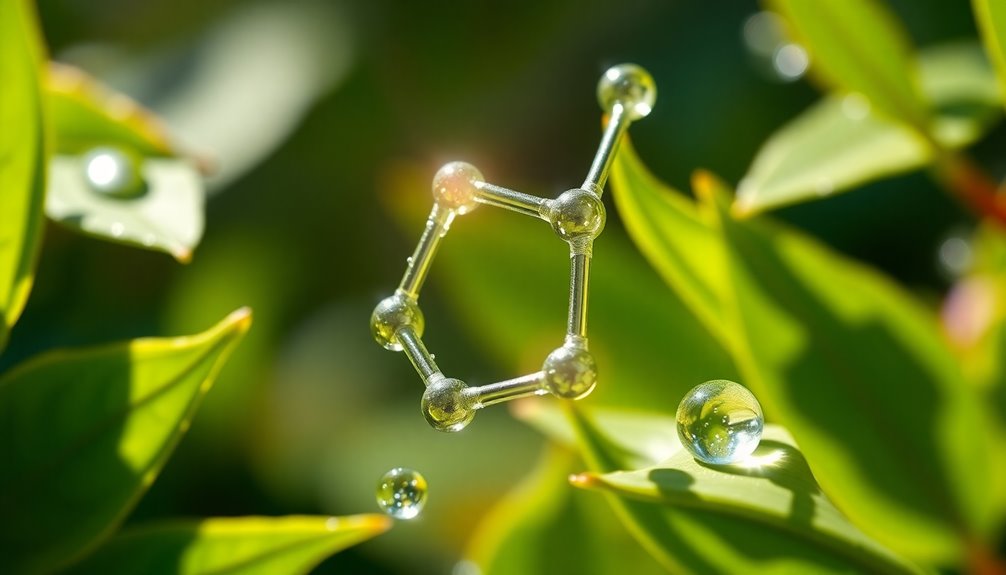
Benzene serves as the quintessential example of an aromatic compound, showcasing the defining characteristics of aromaticity.
With its cyclic structure and six π electrons, benzene follows Huckel's rule (4n + 2) and exhibits remarkable stability due to delocalized electrons. This planar geometry allows for continuous overlap of p-orbitals, enhancing its aromatic nature.
The resonance energy of benzene is a key factor, making it less reactive than many non-aromatic compounds.
Because of its unique properties, benzene plays a significant role in industrial applications, serving as a building block for a variety of chemicals, including dyes, plastics, and pharmaceuticals.
Understanding benzene deepens your appreciation for aromatic compounds and their significance in the chemical world.
Example Analysis of Ethyl Alcohol

Ethyl alcohol, commonly known as ethanol, is a straight-chain alcohol that stands apart from aromatic compounds due to its structural characteristics. Lacking a cyclic structure, it can't form a benzene ring, which is vital for aromaticity. Ethyl alcohol contains a hydroxyl (-OH) group but doesn't exhibit delocalized π electrons or a complete conjugated system, fundamental criteria for aromatic compounds.
Feature | Ethyl Alcohol |
---|---|
Structure | Straight-chain |
Aromaticity | Non-aromatic |
Delocalized Electrons | Absent |
This lack of resonance and linearity is why ethyl alcohol is classified as non-aromatic, making its chemical behavior quite different from that of aromatic compounds.
Example Analysis of Methane

When you look at methane (CH4), you see a simple structure with one carbon atom surrounded by four hydrogen atoms, creating a tetrahedral shape.
It's essential to recognize that methane doesn't have a cyclic structure or double bonds, which means it can't be classified as aromatic.
Instead, its saturated nature and lack of π electrons highlight its non-aromatic characteristics.
Methane's Structure Overview
Methane, the simplest hydrocarbon, showcases a straightforward molecular structure. It consists of one carbon atom bonded to four hydrogen atoms, forming a tetrahedral shape.
This non-cyclic arrangement means that methane lacks the necessary criteria for being classified as aromatic. Aromatic compounds require a cyclic structure and the presence of pi electrons, which methane simply doesn't have.
You'll notice that methane is fully saturated, with no double bonds or alternative bonding configurations. As a result, it doesn't exhibit the unique stability or reactivity typical of aromatic compounds.
Instead, methane is classified as a non-aromatic compound, highlighting its distinct characteristics in the broader context of hydrocarbon structures. Understanding this helps clarify why methane remains outside the aromatic domain.
Non-Aromatic Characteristics Explained
Exploring the characteristics of non-aromatic compounds, you'll find that methane serves as a prime example due to its unique structure.
Methane (CH4) is a linear molecule, which means it lacks the cyclic structure typical of aromatic compounds. With just one carbon atom bonded to four hydrogen atoms, methane doesn't have any pi electrons or double bonds.
This absence of a conjugated system means it doesn't exhibit resonance, a vital feature of aromatic compounds. Additionally, the lack of pi electron delocalization confirms its classification as a non-aromatic compound.
Instead, methane's stability and properties come from its saturated nature, highlighting how non-aromatic characteristics distinctly differentiate it from aromatic compounds in organic chemistry.
Example Analysis of Phenol

When you look at phenol, you'll notice its unique cyclic structure and the delocalized π electrons that give it stability.
This aromatic compound's -OH group affects both its reactivity and water solubility, making it a fascinating subject in chemistry.
Plus, its applications in plastics, resins, and pharmaceuticals highlight its importance in various industries.
Structure and Stability
Aromatic compounds like phenol (C6H5OH) showcase unique structural and stability features that set them apart from other organic molecules. Phenol's cyclic structure and fully conjugated π electron system contribute to its aromatic stability, fulfilling Huckel's rule with six π electrons. This planar structure allows for continuous p-orbital overlap, essential for maintaining aromaticity.
Feature | Description | Importance |
---|---|---|
Cyclic structure | Six-membered ring | Defines aromaticity |
Conjugated system | Fully overlapping p-orbitals | Enhances stability |
Hydroxyl group | –OH influences solubility | Retains aromatic character |
Huckel's rule | Satisfies 4n + 2 with n = 1 | Guarantees aromatic stability |
These factors make phenol a quintessential example of aromatic compounds.
Reactivity in Chemistry
Understanding phenol's reactivity in chemistry reveals how its unique structure influences its interactions with other compounds. As an aromatic compound, phenol features a cyclic structure and a fully conjugated π electron system, which enhances its chemical properties.
Here are three key aspects of phenol's reactivity:
- The hydroxyl (-OH) group makes phenol a stronger acid compared to aliphatic alcohols.
- Its aromaticity contributes to stability, reducing reactivity under certain conditions.
- Phenol readily participates in electrophilic substitution reactions, making it a valuable precursor in organic synthesis.
These characteristics show why phenol is essential in various applications, thanks to its versatile reactivity and the influence of its aromatic nature.
Applications in Industry
Phenol's unique reactivity makes it a valuable asset across various industries. As an aromatic compound, phenol plays an important role in producing plastics like polycarbonate and epoxy resins, thanks to its strong chemical properties.
In the pharmaceutical field, you'll find phenol used as a precursor in synthesizing drugs, including antiseptics and analgesics, highlighting its medicinal importance. Historically, phenol was the first antiseptic used for sterilizing surgical instruments and disinfecting wounds.
Additionally, it contributes to vibrant colors in textiles and coatings through dyes and pigments. Its ability to act as a solvent also makes phenol essential in chemical analyses and organic synthesis processes.
Non-Aromatic and Anti-Aromatic Compounds

While many compounds exhibit aromatic characteristics, some fall outside this category, including non-aromatic and anti-aromatic compounds. Understanding these distinctions is essential in organic chemistry.
Here are three key points to remember:
- Non-Aromatic Compounds: These lack a cyclic structure, resonance, or a fully conjugated π electron system. A classic example is cyclohexane, which, despite being cyclic, has no π bonds.
- Anti-Aromatic Compounds: Characterized by having 4n π electrons, they're cyclic and planar but fail to satisfy Huckel's rule, making them unstable.
- Reactivity: Anti-aromatic compounds, like cyclobutadiene, exhibit heightened reactivity due to their inability to delocalize electrons effectively.
Recognizing these differences helps you predict chemical behavior accurately.
Applications of Aromatic Compounds
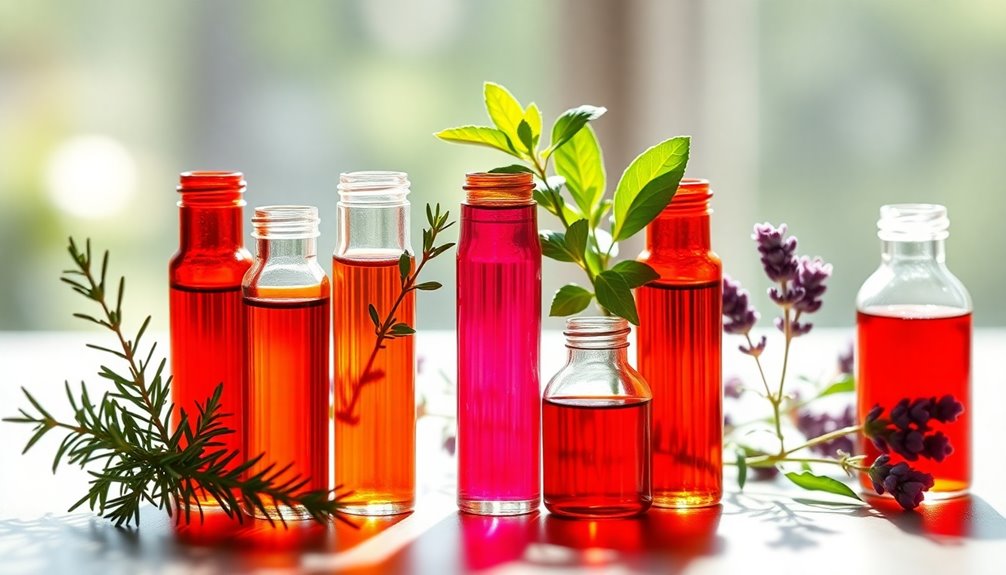
Aromatic compounds are integral to various industries, thanks to their unique properties and stability.
In pharmaceuticals, you'll find these compounds as key ingredients in many medications, leveraging their reactivity for effective treatments. They're also essential in the manufacture of dyes and pigments, where they provide vibrant colors that enhance product appeal.
In the fragrance industry, aromatic compounds serve as vital components in perfumes and scented items, contributing to their desirability.
Furthermore, in industrial applications, you can rely on aromatic compounds as solvents, facilitating various chemical processes due to their ability to dissolve organic substances.
Their unique stability and resonance make them invaluable in chemical synthesis, allowing researchers to create complex organic molecules for development and innovation.
Frequently Asked Questions
Which of the Following Is an Aromatic?
When you're trying to identify an aromatic compound, look for a cyclic structure, planarity, and a fully conjugated π electron system.
Remember Huckel's rule, which states that a compound must have 4n + 2 π electrons to be aromatic. For instance, benzene, with its six π electrons, is a classic example.
If a compound lacks these characteristics, it likely isn't aromatic, so always check the structure and electron count closely!
What Is an Aromatic Example?
Did you know that benzene, the most famous aromatic compound, has six carbon atoms arranged in a perfect hexagon?
When you think of aromatic compounds, benzene's structure, with its alternating double bonds and six delocalized π electrons, immediately comes to mind.
Other examples include toluene, which features a methyl group attached to benzene, and naphthalene, with its fused rings.
These compounds showcase the unique stability and reactivity associated with aromaticity.
Which Are the Aromatics?
When you're trying to identify aromatic compounds, look for those that follow Huckel's rule, meaning they've 4n + 2 π electrons.
You'll find that compounds like benzene, toluene, and naphthalene are classic examples. These compounds are cyclic and planar, allowing for delocalized π electrons, which lends them stability and unique reactivity.
Which Species Are Aromatic?
Did you know that over 60% of modern pharmaceuticals contain aromatic compounds?
When identifying which species are aromatic, you'll look for cyclic, planar structures that follow Huckel's rule, meaning they've 4n + 2 π electrons.
Classic examples include benzene, naphthalene, and toluene.
If a compound disrupts this pattern, like having too few or too many π electrons, it won't be considered aromatic.
Understanding these characteristics is essential for your studies in organic chemistry.
Conclusion
In the vibrant world of chemistry, aromatic compounds are like the dazzling stars in a night sky, enchanting with their unique stability and delightful scents. By understanding the criteria for aromaticity, you can easily spot these fascinating structures among their non-aromatic and anti-aromatic counterparts. So, next time you encounter a compound, let your curiosity guide you—embrace the aromatic journey, and watch as the enchanting beauty of these molecules unfolds before your eyes!